NASCAR heads north this weekend for an action-packed several days of racing at Pocono Raceway, commonly referred to as The Tricky Triangle. The track was originally opened in 1968 as a three-quarter mile short track, but was reconfigured into a two-and-a-half-mile speedway in 1970. The reconfigured circuit was designed by two-time Indianapolis 500 champion Rodger Ward who picked three corners from his favorite racetracks and tried to come up with a way to combine them. Turn 1 is modeled after the now defunct Trenton Speedway’s Turn 4, Turn 2, also known as the tunnel turn, is taken from Indianapolis Motor Speedway, and Turn 3 is based on the Milwaukee Mile.
Pocono Raceway hosted its first Indycar race in 1971 and began welcoming the NASCAR Cup series in 1974. In 1984 the track was granted a second annual Cup series date which it held onto until 2021 after which it was reduced back to one visit every year. The Indycar series removed Pocono Raceway from its schedule for 2020 and beyond after a rash of serious accidents including the 2015 death of Justin Wilson and a 2018 crash that left Robert Wickens paralyzed.
Looking at the circuit from above, you can see that the Tricky Triangle is technically a scalene, meaning that it has three sides of unequal length and three unequal angles, two acute and one slightly obtuse.
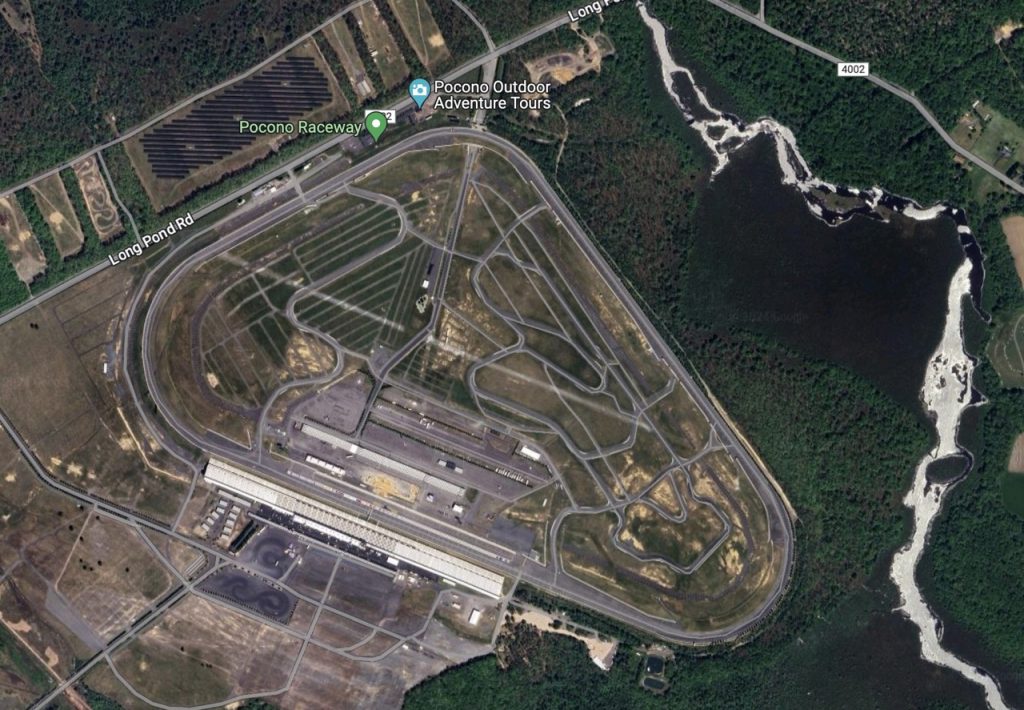
The front straightaway is 3,740ft (1,140m) in length and sends drivers rocketing off into Turn 1 at around 195mph (315kmh). The first corner has a radius of 675ft (206m) and a banking angle of 14* which produces a minimum speed of roughly 145mph (235kmh). The 3,055ft (930m) “back” straightaway connects Turn 1 with the tunnel turn which has a radius of 750ft (228m) and is banked at 8*. Drivers will enter the tunnel turn at around 190mph (305kmh) before decelerating to an apex speed of around 150mph (240kmh). After exiting the tunnel turn, drivers will fly down the 1,780ft (545m) short chute, accelerating up to 180mph (290kmh), before arriving in Turn 3 which has a radius of 800ft (245m) but is only banked at 6* giving an apex speed of about 140mph (225kmh).
Pocono Raceway’s front straightaway is the third longest in NASCAR, 60ft (18m) shorter than Daytona’s backstretch and 160ft (48m) shorter than the Alabama Gang Superstretch at Talladega Superspeedway. The front straightaway is 340ft (103m) longer than the front and back straights of the legendary Indianapolis Motor Speedway. Even the shortest straightaway at Pocono is several hundred feet longer than the backstretch at most intermediate ovals, 1,780ft (545m) compared to 1,500ft (460m) at Charlotte Motor Speedway. The length of these straightaways makes Pocono Raceway into what is essentially the Monza of oval racing where finding a balancing point between downforce and drag is crucial to making lap time.
Any time that downforce is added to a vehicle, there is also a drag penalty to be paid. Downforce is just negative lift and a car’s aerodynamic efficiency is discussed in terms of its L/D ratio, which is the negative lift coefficient divided by the drag coefficient. Formula 1 cars produce some of the highest downforce levels on any modern racing car, but they are also incredibly draggy. When a driver lifts off the throttle in a Formula 1 car, the aerodynamic drag alone produces around 1G worth of deceleration. For comparison, the maximum stopping capability of a traditional road car is around 0.6 to 0.8G.
Go High Or Go Low?
Arguments can be made for either case, low downforce or higher, so let’s examine them. Of a roughly 54.5-second lap around Pocono Raceway, drivers will spend about 30.8 seconds, or 57% of the lap, at wide open throttle. When travelling in a straight line at wide open throttle the air is literally the only thing holding the driver back from going faster. Essentially, for 57% of the lap, a car’s speed will be drag-limited and for the remaining 43% it will be downforce-limited. A car built with less downforce will be forced to navigate each corner at a slightly lower speed than one built with more downforce.
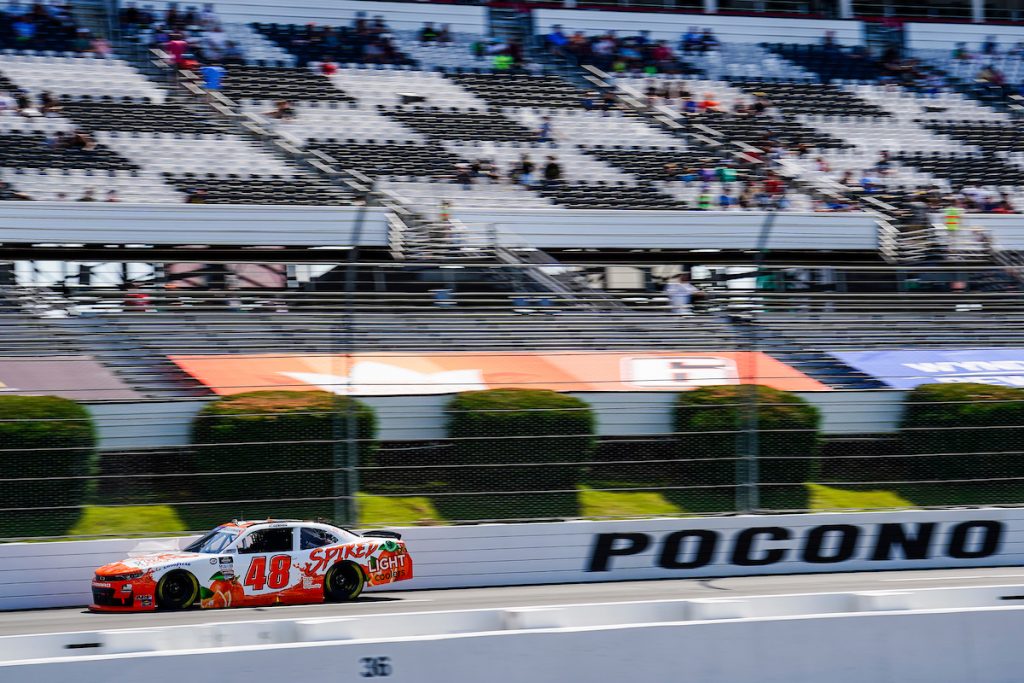
The advantage of a low downforce setup is that the car will have a higher terminal velocity and will also be able to accelerate at a faster rate. A car that is built with more downforce will navigate the corners at a higher rate of speed, but will have what is essentially a boat anchor holding them back down the straightaways, limiting both its top speed and its rate of acceleration. Finding the point of diminishing returns for both downforce and drag is one of the team’s primary goals.
Between these massive straightaways we’re left with three corners that behave completely differently to one another. Turn 1 is the steepest bank with the highest entry speed. Turn 3 is the flattest, has the lowest entry speed, and is also the longest. For more information about how banking and corner speed affects the aero platform of a car, check out my piece on Texas Motor Speedway. Each corner also has a different angle of rotation as well with Turn 1 being the sharpest and Turn 2 being the most open.
Turn 3 leads onto the longest straightaway on the circuit so it is crucial to nail. Any understeer that causes a driver to wait on the throttle exiting Turn 3 will cost significant lap time. Unfortunately, building a car that rotates well in the long, slow and flat Turn 3 will leave you with one that feels very unstable in the faster and more banked Turn 1. Drivers will often talk about both ends of the circuit feeling very disconnected. The holy grail of setting up a car for Pocono Raceway is to build one that is both secure in Turn 1 and doesn’t understeer in Turn 3.
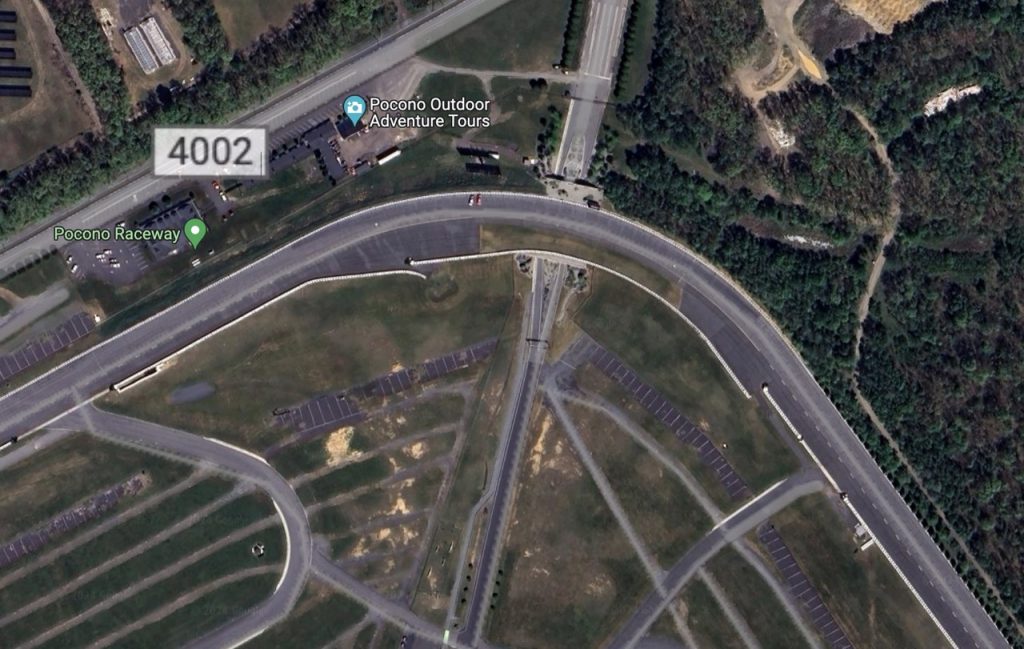
Turn 2, aka the tunnel turn, may very well be the most difficult turn on a NASCAR oval. Looking at the racetrack from above you can see that the infield entrance tunnel runs directly under the center of the corner.
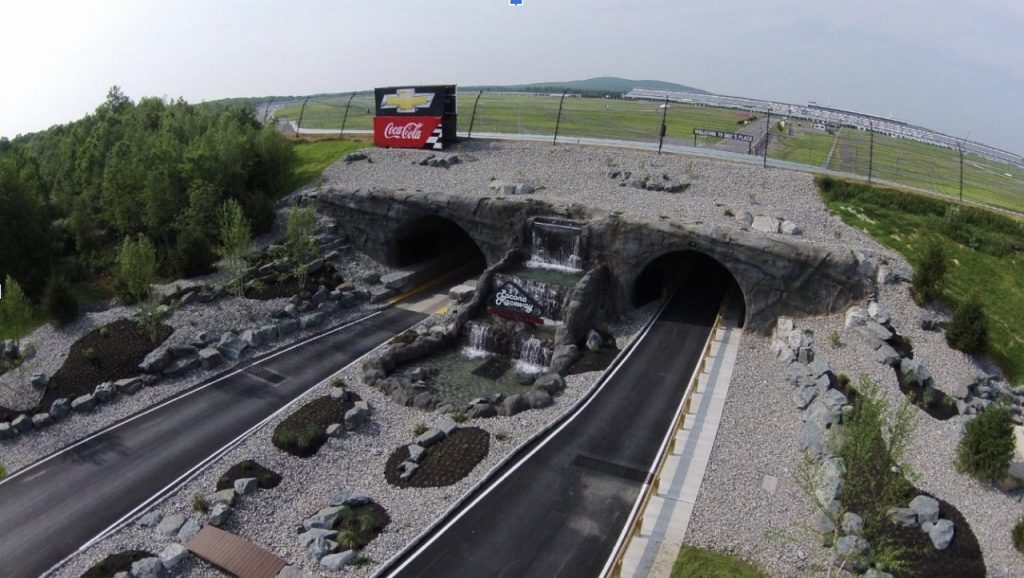
So why does this tunnel make things tricky for drivers? After a racetrack is paved, the weight of the asphalt causes the track surface to sink into the ground slightly over time. This is especially prevalent in the corners where the banking is initially created by piling and packing dirt. That’s all well and good, but when a tunnel is placed under the racetrack suddenly there is an area that cannot sink. The concrete structure of the tunnel is rigid underneath the asphalt. When the rest of the track settles into the banking, one section becomes slightly raised. Tunnels also cause the racing surface to age in a weird way. Asphalt that is laid over dirt is kept more thermally stable through the seasons by the earth underneath it acting as a heat sink. The asphalt that is laid over the tunnel experiences bigger swings in temperature as it is sandwiched on both sides by the ambient air temperature. This creates a transition region on both sides of the tunnel where the asphalt is expanding and contracting at different rates and over the years more cracks will develop in this area. You can see below as Austin Hill navigates the tunnel turn just how much these bumps unsettle the car’s suspension. That camera shot also provides a good visualization of how the cars are skewed out while cornering. The left front tire is on the yellow line while the left rear tire is a foot or so above it.
As we saw from the aerial shots, the tunnel turn is more of a kink than an actual corner, think Turn 11 at Road America, and as such it has the highest apex speed. Nailing Turn 2 requires drivers to send their car off into the corner hard and flick it in with as little off-throttle time as possible. It takes both maximum commitment and utmost precision to get it right.
Drivers will encounter the tunnel bumps just as they are trying to go back to full throttle. You can see below how these bumps caused Kyle Larson to crash during practice for the 2019 Cup series race. In the onboard footage you can hear the car bottom out slightly which causes the rear of the car to oversteer and Kyle is never able to recover. From the external shots you can see sparks coming out from under the right rear of the car. This was more than likely the bottom of the car’s track bar mount striking the racetrack as the rear suspension compresses over the bumps.
In the Craftsman truck and ARCA series, it is possible to navigate this corner without lifting completely out of the throttle. Drivers consider it a badge of honor to be able to flat-foot the tunnel turn on sticker tires. In 2015 we were testing here with then rookie Kyle Weatherman. Trevor Bayne was slated to drive the race as Kyle was too young, but had a wedding to attend on the day of the test. Trevor stopped by the track in the morning to put up a lap time before we swapped drivers. Kyle got in the car and was immediately quick. Each lap he picked up a tenth or two before matching Trevor’s lap time on his fifth circuit. On lap six Kyle tried to flat-foot the tunnel turn for the first time and backed the car into the fence.
Pocono’s three corners are difficult enough, but the length of the straightaways punishes even the smallest mistake exponentially. Drivers will enter Turn 1 travelling at roughly 290ft/sec (90m/sec) and must spot their braking and turn-in markers with pinpoint accuracy. The slightest mistake will lead to massive lap time loss.
Once the driver is back to full throttle there is nothing that they can do to accelerate faster in order to make up for a mistake. If they are at a deficit leaving the corner it will result in them bleeding lap time all the way until the beginning of the next corner.
Due to its unique layout, Pocono Raceway is just as hard on racecars as it is on drivers. Tire failures at Pocono Raceway are another side effect of the long straightaways. The Cup series will be using the Goodyear 5186 left-side tire compound this weekend. This tire is also utilized at Las Vegas Motor Speedway and several other intermediate ovals. So why is this an issue? Let’s take a look.
It’s All About Tires
A lap around Pocono Raceway is 64% straightaway, whereas Las Vegas Motor Speedway is only 46% straightaway, which is comparable to other intermediate tracks. As a car’s suspension compresses and rolls into a corner, the camber angle of the front tires will change. Each tire has a specific camber angle at which it will generate the most grip when cornering.
In order to achieve this angle in the corners after the suspension compresses and rolls, the static, or straightaway camber, is offset by several degrees. The change in camber angle relative to suspension movement is what’s known as a car’s camber curve. To understand this concept, let’s start by looking at a generic view of an oval track front suspension. The left front tire has positive camber, meaning the top is leaned out, and the right front tire has negative camber, meaning that the top is leaned in.
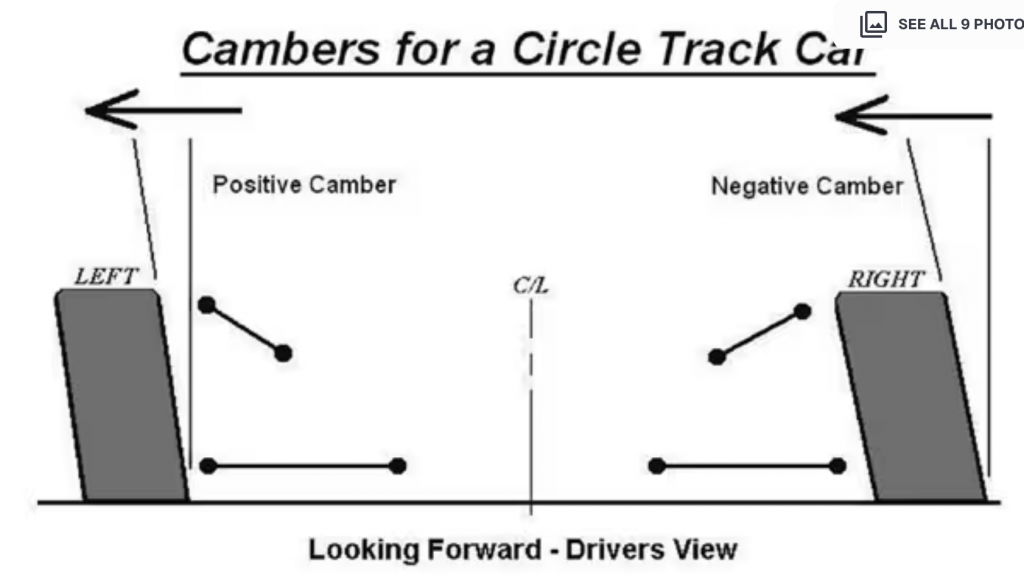
Let’s isolate the left front suspension for a moment. By connecting an imaginary line between the upper and lower control arms we can create what’s known as a front view swing arm, or FVSA. The FVSA acts as the imaginary arm about which the suspension rotates through its instant center. From the diagram below you can see that as the tire travels up relative to the chassis, the top of the tire would be pulled in. On a circle track car this would reduce positive camber and cause the tire to stand up closer to vertical.
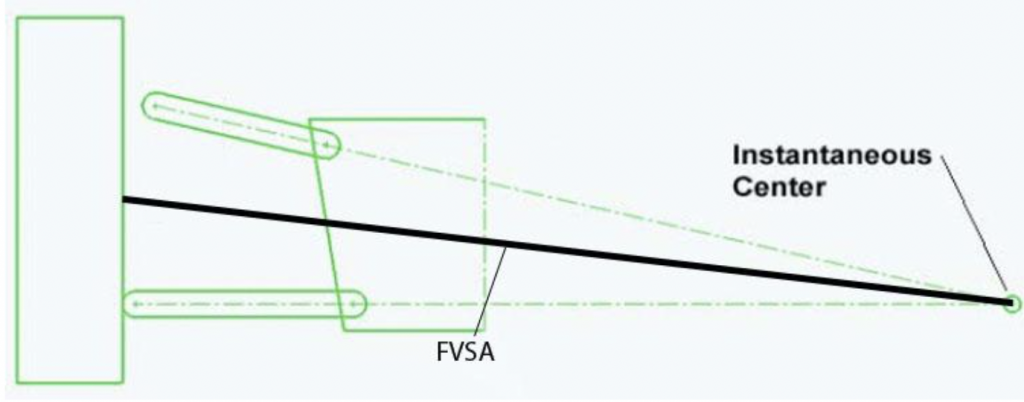
The rate of camber change relative to suspension travel is dependent on the length of the FVSA. A shorter FVSA means that the camber angle will change faster and a longer FVSA means that the camber angle will change slower.
Because the left front tire wants to stand up, or lose camber, in travel the suspension will be designed to have more positive camber on the straightaways. This effectively causes the tire to ride on its edge when the car is not cornering. Riding on the edge of the tire causes increased deflection of the tire’s sidewall. Imagine you have a paperclip in your hand. You should be easily able to unroll the paperclip and straighten it out without damaging it. However, if you take the paperclip and bend it repeatedly back and forth several times, it will snap in half.
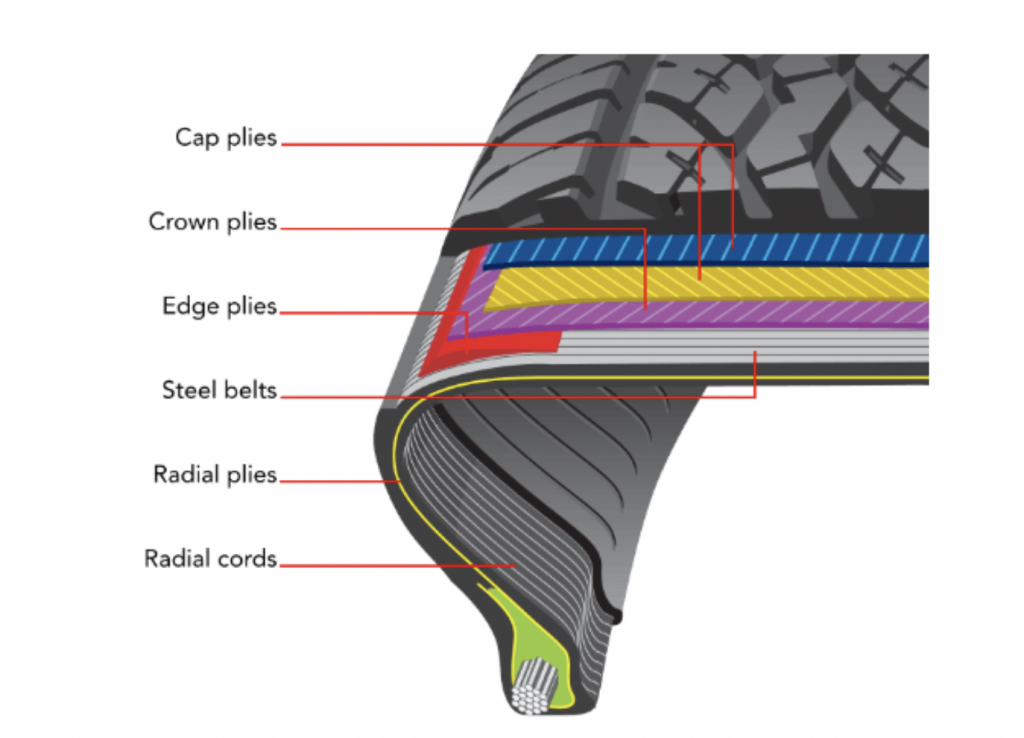
This is what happens to the metal bands in a tire’s sidewall when it experiences too much deflection. Every time the tire completes a revolution it is like bending the paperclip one more time. Eventually, it will snap and the tire will fail. This phenomenon is what caused Kyle Larson’s left front tire to fail entering the final corner of the final lap while he was leading the 2021 Cup series race at Pocono.
If a team was worried about their left front tire, they could achieve the same dynamic camber by altering the FVSA length to reduce the required static camber. This can be done in a number of ways such as changing suspension pivot heights or upper control arm length.
For more information on front suspension geometry calculations, you can check out this excellent video by Vatsal Jain as he walks through most of the derivations in depth.
Brake failures are another problem unique to and especially prevalent at Pocono Raceway. Outside of short tracks and road courses, Pocono is easily the hardest racetrack on a car’s brakes. The problem here is once again related to the length of the straightaways. After releasing the brake pedal on entry to Turn 3 it will be approximately 17.5 seconds before the driver jumps back onto the brakes for the entrance to Turn 1. Between Turn 1 and Turn 2 it will be another 16 seconds. Even the short chute between Turns 2 and 3, drivers will be off the brake pedal for just over 9 seconds. You can watch Kasey Kahne’s footwork below during the 2017 Cup Series race at Pocono Raceway below.
The issue here isn’t the amount of braking being done but the time in between braking events. Whenever a driver is not using the brake pedal, air is being forced in through the car’s cooling ducts, reducing the temperature of the braking system. At a normal racetrack where braking events are spaced closer together a team’s biggest concern is managing to get enough air into the system to keep the brakes from overheating. At Pocono, it’s the opposite. Brake cooling must be carefully controlled so as not to cool the brakes too much down these long straightaways.
It is common knowledge that metal expands as it is heated and contracts as it is cooled. If the brakes are being over-cooled, the contraction on each straightaway and rapid expansion under braking will cause stress fractures to develop in the brake rotors which reduce their structural integrity. Eventually, the driver will go to stand on the brake pedal for a corner and the force of the brake pads clamping onto the rotor will cause it to shatter and the driver will be sent hurtling into the wall at nearly 200mph. You can see below where this happened to Jimmie Johnson during a 2017 Cup series race. As he attempted to brake for Turn 1, the right rear brake rotor failed. The smoke you see is the result of brake fluid coming out of the damaged caliper and catching on fire.
Just remember this next time you’re racing around a scalene triangle.
Photos: Author, Daylon Barr/Big Machine Racing